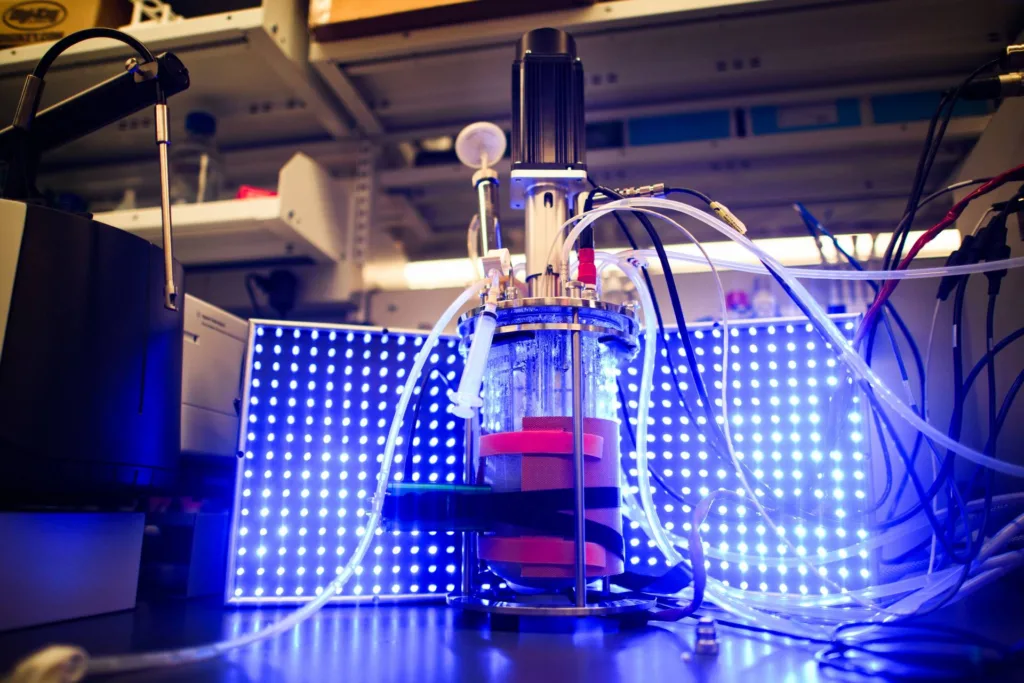
The yeasts targeted by CABBI Conversion researchers can be used to convert plant material into sustainable biofuels and biochemicals. And through the use of genetic engineering with the help of a phase II CABBI PI, these yeasts can be dynamically controlled by light.
José Avalos, a recent addition to the CABBI Conversion Theme, is using light to study and optimize the growth and productivity of yeasts. Avalos is an Associate Professor of Chemical and Biological Engineering at Princeton University. He is also appointed to the Andlinger Center for Energy and the Environment and the Omenn-Darling Bioengineering Institute at Princeton; and most recently the recipient of the 2022 ACS-BIOT Young Investigator Award.
“I’m very excited to be a part of CABBI,” Avalos said. “This community has many very smart researchers, and we all have a common goal. I get a lot of satisfaction thinking that my work can contribute to helping us move beyond petroleum. Anything that our work can contribute to that end is what motivates me.”
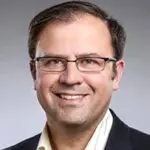
An expert in molecular engineering and synthetic biology, Avalos was specifically recruited for his ability to engineer dynamic controls into organisms. These dynamic controls mean that researchers can easily and reversibly turn on and off genes that influence an organism’s growth and productivity at different times, strengths, and for different durations.
Some yeasts naturally produce chemicals of interest as they grow. A classic example of this is how baker’s yeast produces ethanol. Researchers don’t need to engineer the baker’s yeast to first grow and then produce, because it already produces as it grows.
However, when researchers engineer yeasts to produce something they don’t normally produce, that often slows down the yeasts’ growth. This can happen for many reasons, but one of the key issues is that the metabolic resources that the yeast normally uses to grow are being diverted to produce something that the cell doesn’t need. This is a significant problem because the overall productivity of the cell will go down if it takes a long time to grow.
Having dynamic controls enables two-phase fermentation: the cell first grows without producing a chemical that is unnecessary for its growth, and then switches to producing that chemical once it’s fully grown. By first growing and then producing, the yeast can be made much more productive.
Avalos’s team brings an exciting and unique method of dynamic control to the table: optogenetic engineering, in which cells can have certain genes turned on or off in response to light.
“We can essentially direct a cell to either grow or produce, depending on what kind of light, if any, we’re shining on them,” Avalos said. “Dynamic control is typically done with chemicals, but using light instead has some big advantages. Switching from growth to production with a chemical is hard to reverse. It’s not easy to just take away the chemical. But it’s very quick and easy to turn a light on or off. With light, the two phase fermentation can be turned into any number of phase fermentation.”
Optogenetic controls are engineered into an organism by borrowing proteins from nature. There are many organisms in the natural world that respond to light: many plants move towards light, seeds germinate in response to infrared light, and cyanobacteria manage to float in the ocean by changing their buoyancy in response to light. The genes and proteins from nature that are responsible for these responses to light can be implemented in yeasts for optogenetic controls.
The ability to quickly and reversibly turn genes on and off also allows Avalos’s team to carefully study the flux across metabolic pathways in the cell.
“If you imagine this flux analysis as being a network of pipes, we’re trying to measure how much water each pipe holds,” Avalos said. “Having this type of dynamic control with light allows us to open or close the valve to one of the pipes almost instantly. And then you can measure how all the other pipes readjust. That’s a type of study that we haven’t done yet, but we’re looking forward to doing it with CABBI researchers.”
By understanding the dynamics of their metabolic pathways, this kind of study would help scientists optimize the growth and production of the yeasts they work with.
Avalos’s team is specifically studying the use of optogenetics in Issatchenkia orientalis for citramalate production. I. orientalis is a yeast renowned for its extraordinary ability to tolerate many stressors, ranging from low pH to fungicides. Because of this, I. orientalis is thought to be a promising option for the sustainable production of organic acids, such as citramalate, at an industrial scale. Citramalate is a precursor to valuable compounds that are used in everyday products. This organic acid can be upgraded to lightweight, transparent, shatter-resistant materials, which are used in eyeglasses, phones, and other electronics.
To achieve optogenetic control in I. orientalis, Avalos’s team is using a transcription factor that turns genes on when exposed to blue light. They’re working to implement additional transcription factors in I. orientalis in the future, that will respond to red, green, or infrared light. The more colors of light the organism responds to, the more different genes the researchers will be able to switch on or off independently of each other. This will allow the team to study more different combinations of gene activity.
“Something really exciting about this research is that this can be applied to any of these pathways and any of these cells,” Avalos said. “I like to think about what kind of problems could be addressed with our capabilities in other systems beyond Issatchenkia for citramalate.”
In addition to Avalos, Chris Gonzalez and Krishi Mantra, both Chemical and Biological Engineering Ph.D. Candidates, and Jaewan Jang, a Postdoc, are also hard at work on optogenetically engineering I. orientalis for citramalate and possibly 3HP production. Jeremy Cortez, a Molecular Biology Ph.D. Candidate, is also exploring the use of genetically encoded biosensors for chemical production in screens implemented in CABBI’s iBioFAB biofoundry.
To learn more about these researchers and the exciting work their team is doing, check out the Avalos Research Group website.
— Article by CABBI Communications Specialist April Wendling